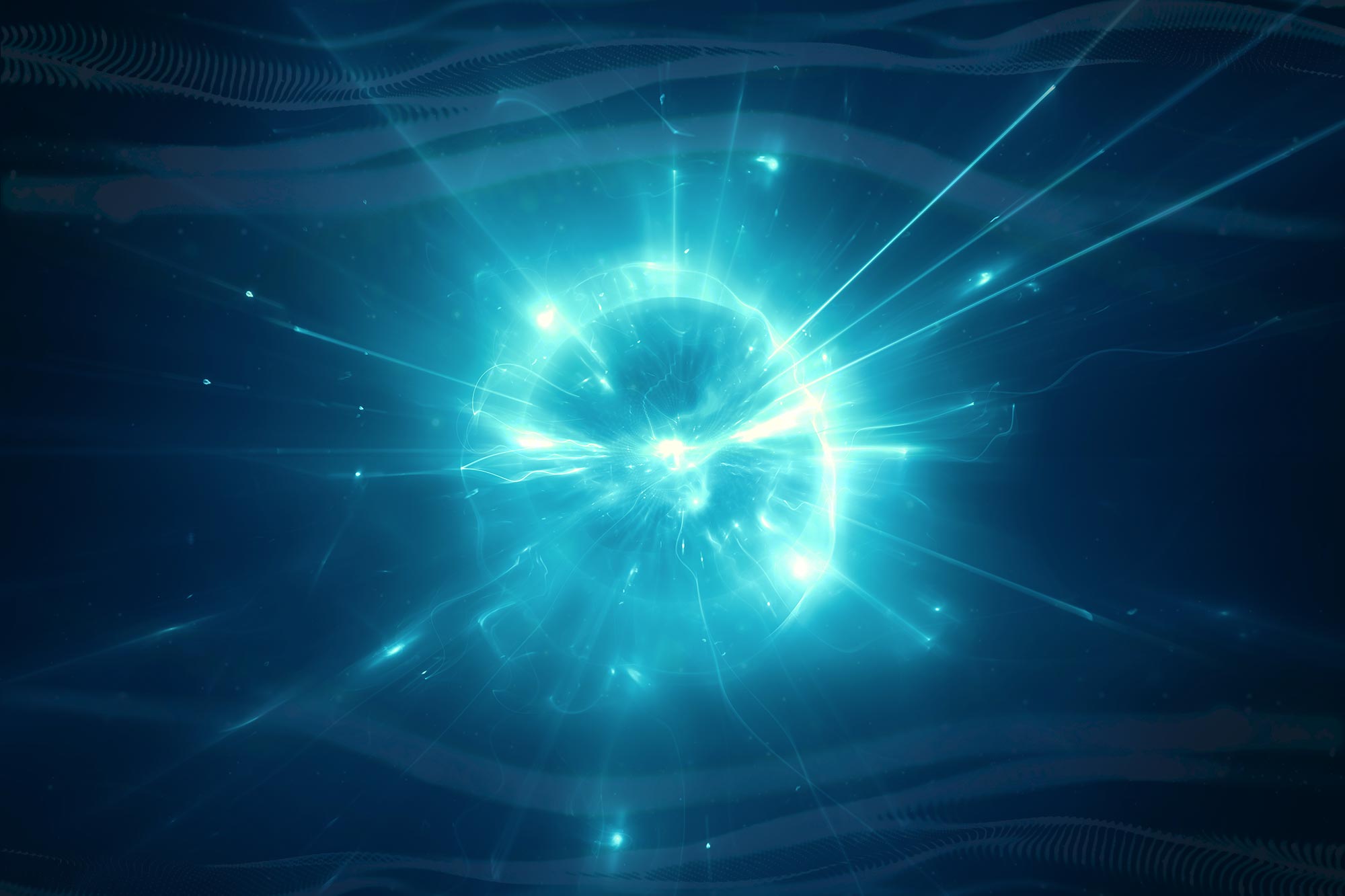
This illustration represents a dark matter particle. Scientists at Oak Ridge National Laboratory have attempted to monitor these elusive particles using neutrino detectors in Neutrino Alley.
Scientists at Oak Ridge National Laboratory attempted to observe dark matter in a brightly lit basement corridor using the sensitivity of their neutrino detectors. Neutrino Alley, where the team is working, is located below the Spallation Neutron Source, a powerful particle accelerator. After years of theoretical computation, the COHERENT team set out to observe dark matter, which is thought to make up up to 85% of the universe’s mass. The experiment allowed the team to broaden the global search for dark matter in a new way, and they plan to receive a larger, more sensitive detector to improve their chances of catching dark matter particles.
Few things carry the same aura of mystery as dark matter. The name itself radiates secrecy, suggesting something hidden in the shadows of the universe.
Called a collaborative team of scientists coherentincluding Kate Schulberg, Distinguished Professor of Physics of Arts and Sciences, Philip Barbeau, Associate Professor of Physics, and postdoctoral researcher Daniel Berchie, have tried to bring dark matter out of the shadows of the universe to a less glamorous destination: a brightly lit, cramped basement hallway.
Not an ordinary cellar, though. Working in an area of Oak Ridge National Laboratory nicknamed Neutrino Alley, the team usually focuses on subatomic particles called neutrinos. They are produced when stars die and become supernovae, or on a level closer to Earth, as a byproduct of colliding protons in particle accelerators.

Dark matter, the invisible stuff that makes up 85% of the matter of the universe, isn’t hidden far between galaxies. A team of scientists is trying to bring her out of the shadows. Credit: X-rays: NASA/CXO/Fabian et al. ; Radio: Gendron-Marsolais et al. ; NRAO/AUI/NSF Optical: NASA, SDSS
Not coincidentally, Neutrino Alley is located directly under one of the world’s most powerful particle accelerators, Oak Ridge’s. Spallation neutron source (SNS). Neutrino Alley houses a collection of detectors specifically designed to monitor neutrinos as they pass by and collide with them.
However, neutrinos are not the only by-product of SNS processes. Dark matter (not to be confused with the villain’s favorite antimatter) is also produced when particle accelerators smash protons together. After years of theoretical computation, the COHERENT team set out to harness the power of SNS and the sensitivity of their neutrino detectors to monitor dark matter in Neutrino Alley.
“And we haven’t seen that,” Schulberg says. “Of course, if we had seen it, it would have been more exciting, but not seeing it is actually a big problem.”
She explained that the fact that dark matter has not been detected by her neutrino detectors allows her to improve theoretical models of what dark matter looks like.
“We know exactly how a dark matter detector would respond if the dark matter had certain properties, so we were looking for that specific signature.”

Kate Schulberg, co-author Grayson Rich and Philip Barbeau. Credit: Long Li/Duke University
The fingerprint in question is the way the nuclei of the atoms in the neutrino detector bounce off when they hit a neutrino, or in this case, a dark matter particle.
“It’s like throwing projectiles at a bowling ball on a piece of ice,” Berchie said. Bowling balls, in his analogy, are the atoms in the neutrino detector – which in this experiment was a 14.6kg cesium iodide crystal. “You can tell a lot about the sling and the force you throw by how high the bowling ball bounces on contact.”
When it comes to dark matter, any information is good information. Nobody knows what it really is. Almost 100 years ago, physicists realized that the universe could not behave the way it did if all it contained were the things we could see.
“We’re swimming in a sea of dark matter,” said Jason Newby, head of the Neutrino Research Group at Oak Ridge National Laboratory and co-author of the study. The consensus of physicists is that dark matter makes up 85% of the mass of the universe. It must be subject to gravity to explain the behavior of the universe, but it does not interact with any kind of light or electromagnetic waves, and appears dark.

Jason Newby and co-author Yuri Efremenko carry the very small 14.6 kg cesium iodide neutrino detector used to search for dark matter at Neutrino Alley. Credit: Genevieve Martin/Oak Ridge National Laboratory, US Department of Energy
“We learned this by looking at large galaxies that orbit each other, and we saw that they rotate way faster than they should, which implies that they have more mass than they appear to be,” Birchi said. “So we know there are additional things out there, we just need to know where to look for them.”
“Even though most of the time we don’t get results, it’s really important that you look everywhere and then you can rule out a whole number of possibilities and focus on a new area with strategy rather than just using the ‘spaghetti on the wall’ approach,” said Newby.

Daniel Berchi. Credit: Duke University
“We’re extending our reach to any models that could exist for dark matter, and that’s very powerful,” said Schulberg.
And the achievement doesn’t stop there, she notes: The experiment also allowed the team to broaden the global search for dark matter in a new way.
“The typical detection technique is to go underground, build a very sensitive detector, and wait for the dark matter particles to pass by,” Berchi said.
the problem? Dark matter particles may be traveling quietly through the air. If it is also very light, it may not reach the detector with enough power to create a detectable fingerprint.
The COHERENT team’s experimental setup addresses this issue.
“When you go into the accelerator, you produce those particles at significantly higher energy,” Berchi said. This gives them a lot gravity to hit the cores and show the dark matter signal. “
So what now? It’s not quite back to the drawing board. Neutrino Alley is currently preparing to receive a larger, more sensitive detector, which, along with COHERENT’s refined search criteria, will greatly improve the chances of catching one of these demon particles.
“We’re at the threshold of where dark matter needs to be,” Birchi said.
Reference: “First Sub-GeV dark matter probe beyond cosmological predictions using the COHERENT CsI detector in the SNS” by D. Akimov et al. February 3, 2023 Physical review letters.
DOI: 10.1103/PhysRevLett.130.051803
More Stories
Watch a Massive X-Class Solar Explosion From a Sunspot Facing Earth (Video)
New Study Challenges Mantle Oxidation Theory
The theory says that complex life on Earth may be much older than previously thought.